2020. 3. 4. 08:29ㆍ카테고리 없음
Geotechnologies and related science and engineering fields make it possible to use underground space to support livable, resilient, and sustainable cities. Geotechnical applications have supported the design and construction of underground facilities, and will continue to be critical to the delivery of underground facilities with lower initial costs and risk, and better lifecycle performance. To contribute to a more resilient and sustainable future, geotechnology will need to more closely integrate the many disciplines related to site investigation, design, construction, operation, and risk management of underground facilities. A better understanding of the sustainability of underground use—for example, minimizing deterioration, increasing resilience, making holistic decisions concerning subsurface hydrogeologic and thermal environments—also will be necessary.
Improvements in underground technologies have enabled great strides in urban development in recent decades, but the complexity and unpredictability still inherent in underground construction are indications that many challenges remain.This chapter provides a brief overview of the state of the art in various technologies that support underground construction and facility operation. Highlighted are technologies that provide opportunities for significant improvement in the delivery of cost-effective lifecycle performance for underground facilities, contribute to improvements in underground space usage, and contribute to resilient and sustainable urban solutions. Technological innovation can advance engineering practice and increase the appeal of underground space. Technological and engineering advances have always been crucial to efficient and economical underground development. Many technological developments have been motivated by practical challenges encountered during construction of a project (e.g., the development of the tunnel shield by Brunel), and the tunneling industry has contributed to or been instrumental in many of these. The highly automated modern tunneling boring machine is an example of an an industry led development as are water proofing and ground improvement technologies that have been introduced and popularized.
In close partnership with academia, industry has developed many analysis and design tools (e.g., finite element analysis methods).Since the time of the Pharaohs, tunnels have been built by cut-and-cover construction methods (El Salam, 2002). The invention of the tunnel shield—which supports unlined ground to reduce the risk of collapse, Sir Marc Isambard Brunel and his son Isambard Kingdom Brunel were able to excavate a tunnel under the Thames River (London) between 1825 and 1843 (Muir Wood et al., 1994; Skempton and Chrimes, 1994) (see for a drawing of Brunel’s shield).
Previous projects involving tunnel boring in soft, saturated soils had been extremely difficult or impossible to complete. The tunnel created an important connection between the north and south banks of the Thames that is still in use almost 170 years later. The application of this new technology heralded the era of shield tunneling.Electrically powered locomotives ushered in the era of modern subway systems around the turn of the twentieth century. Electrification alleviated concerns about hazardous diesel or coal fumes and allowed long-distance underground train travel. Innovations in large-scale ventilation systems permitted underground roadway development.
Climate control systems, improved lighting, and more effective signage made the underground environment more hospitable, comfortable, and appealing for retail functions and mass transportation. Advances in materials technology, computer science, robotic construction technology, and laser guidance have allowed improved subsurface excavation using modern slurry shield and earth pressure balance boring machines (Figure 6.2) and rock tunnel boring machines (Figure 6.3). Those technologies made it feasible to construct tunnels exceeding 50 kilometers in length and at diameters approaching 20 meters, and to tunnel under challenging geologic conditions (e.g., in soft flowing ground or highly fractured rock under high ground and water pressures). Ground modification technologies—e.g., injecting cementitious agents to strengthen and reduce permeability of soil and rock, or temporarily freezing of water-bearingSlurry shield and earth pressure balance boring machines for boring in saturated soils are designed to withstand water under pressure. FIGURE 6.1 Brunel’s tunnel shield.
Marc Isambard Brunel developed this tunnel shield technology to build the first subaqueous tunnel beneath the Thames River (1821-1825). Brick walls were built at the faces of the tunnel and held in place while alternate shields were pushed forward 6 inches. The completed tunnel was 38 feet wide and accommodated two carriageways. SOURCE: (accessed June 27, 2012). Public Domain.FIGURE 6.2 Cross-section of earth pressure balance tunnel boring machine. This tunneling technology is ideal for homogenous soft soils. A screw conveyor is used to transport spoil from the face and helps to control pressure with the coordinated advancement of the machine.
The excavation chamber is filled to support the face and allow the machine to be reactive to earth and groundwater pressures. FIGURE 6.3 Cutter head of a rock tunnel boring machine used to excavate the Chattahoochie tunnel.
Disk cutters cut grooves approximately 4 inches apart in this example. Cording.materials (Figure 6.4)—broadened the geologic and hydrologic conditions under which underground construction may occur. Horizontal directional drilling revolutionized installation of many utilities and greatly reduced the need to close streets to traffic and disrupt life in urban situations.Many of the technologies described above led to changes in engineering practice, and in some cases, to new paradigms in urban planning. Similarly, today’s engineering and technology developments will be crucial to an economically constructed, functional, attractive, energy efficient, and sustainable urban environment. This chapter is grouped under the following themes:.
technologies for underground site characterization, including geologic setting, rock and soil properties, and existing underground infrastructure;. technologies for design and analysis for underground technologies;.
technologies for construction of underground space;. technologies for effective asset management; and. technologies that promote sustainability and resilience.These themes are not necessarily sequential or independent. Observations made during the application of each may inform decision making during any phase of development or operation. Infrastructure design may identify further site characterization needs, and unanticipated conditions encountered during construction. FIGURE 6.4 Artificial ground freezing is applied in the excavations extending under the Danube River.
Freezing groundwater around an excavation improves the load carrying capacity of the soils and provides temporary support during construction. (Left) A crosssectional diagram with the locations of the freezing pipes indicated. SOURCE: IMWS, 2009. Reprinted with permission. (Right) Photograph showing excavation with freezing pipes in place. SOURCE:.may require revisions in design. Monitoring and characterization ideally should occur throughout the infrastructure life cycle.
Observations can lead to a type of informed decision making called observational method (e.g., Peck, 1969; Institute for Civil Engineers (Great Britain), 1996) that can improve economy and safety. Many geotechnical engineers refer to the framework for this method originated by Peck and described in a publication by Nicholson and others (1999).The discussions within each theme are illustrative of technologies in use or that have significant potential for the future. By their nature, disruptive technologies are difficult to anticipate, but can fundamentally shift the way underground space is developed and used. Many of the technologies described in this chapter depend on the use of the observational method for effective decision making.
Suggested are technologies for analyses that allow improved application of the observational method.Engineering urban underground space requires detailed knowledge of the underlying geology and the geologic and human-development histories of a site, alignment, and adjacent areas that may affect or be affected by proposed development. Better subsurface characterization supports better decision making. Unanticipated ground conditions may allow optimized design and more judicious use of resources during construction. Detailed understanding of how the site relates to the broader natural and urban systems allows more complete understanding of the existing engineering challenges and informs underground infrastructure locations and alignments, design, choice of construction methods and tools, and long-term operation of the facility and adjacent structures.Site characterization activities often begin through study of existing data and published information. Currently, relevant information must be gathered from many sources and may not provide adequate—or accurate—information about the geological setting or existing underground structures. Ongoing advances in computational capabilities (e.g., massive database systems, data mining) and georeferencing of data (e.g., survey-grade global positioning systems and geographical information systems) could be of great use in the future.
These issues are further explored later in this chapter.Characterizing Geology, Geologic Material Properties, Contamination, and Natural HazardsA geologic perspective in site characterization is necessary to appreciate, quantify, and manage uncertainties in and variability of soil and rock properties and behavior (e.g., composition, stress-strain behavior, permeability, abrasivity, thermal conductivity) and groundwater conditions, ultimately minimizing costs or avoiding overly conservative design (NRC, 2006; FHWA, 2009). Preliminary field and laboratory work supports preliminary project design and construction planning, but further characterization narrows uncertainties and provides detail on important geologic features (e.g., boundaries of geologic units, fault zones), supports design of specific underground works (e.g., shafts, rooms), and provides information related to other special requirements (e.g., avoiding environmental contamination or in situ stress evaluation). The natural underground environment is inhomogeneous, anisotropic, and highly variable over short spatial extents. Large volumes of geologic material often must be characterized in great detail to detect stratigraphic changes and discontinuities important for predicting ground response to construction. A broad range of invasive and noninvasive technologies and tools are available to carry out in situ field investigations (see for example, ), but existing assessment tools cannot provide complete spatial coverage, accurate zonation, and in-situ material properties.At a project scale, hazardous materials encountered during underground construction can add large and unexpected costs to a project and delay project delivery. Characterizing natural and anthropogenic hazardous materials (e.g., chemical contamination and radiation) and their effects on the natural and built environments for particular construction and operation activities is vital.
Understanding any hazardous materials that may be released or transported as a result of construction and operation is important to long-term sustainability and resilience. Similarly, identifying adequate design approaches to protect underground infrastructure from natural hazards such as earthquakes or floods is critical to a resilient and well-functioning underground facility. Because these topics have a broad connection to sustainability and resilience, their characterization is discussed later in the chapter.Environmental concerns that extend to underground storage and disposal also need to be considered. For example, society is grappling with the risks associated with the emerging technology of carbon dioxide sequestration. As more largescale sequestrations are planned, the need to examine their potential impacts on the ability to develop underground space becomes even greater, because, for example, carbon dioxide could seep into underground space.
Therefore, the solution of one problem could inadvertently result in another problem. A recent NRC report explored the risk associated with induced seismicity as a result of carbon capture and storage and makes specific research recommendations related to, for example, factors other than pore pressure that influence seismicity, and development of physiochemical and fluid mechanical models for carbon dioxide injection into potential underground storage reservoirs (NRC, 2012).Choice of characterization tools depends on a number of factors including depth of interest and ground conditions (e.g., soil versus rock; saturated versus partially saturated). Both traditional in situ technologies (e.g., direct measurement) and noninvasive technologies (e.g., geophysical) can be used to characterize natural and manmade features. Some construction sectors provide guidance on site characterization technology choices through extensive lists of tools and techniques (e.g., FHWA, 2009).
Training and experience in the proper use of the tools, however, is usually as critical as the choice of technology itself.Invasive TechnologiesIn situ testing tools provide direct physical measurement of material properties. In soils, for example, standard penetration tests and cone penetrometer tests (e.g., electric, piezocone, and seismic tools) are used to sample or test soil layers directly by drilling or thrusting sampling tools into the ground. Rock sampling and testing can be borehole based or conducted on the removed core. Borings are used to characterize properties such as soil strength, stiffness, dynamic shear wave velocity, and groundwater properties and quality, and the geology at the borehole location. An individual boring may or may not represent the subsurface only a short distance away given the potential variation in geology.
Directionally inclined and horizontal boreholes and oriented probing tools also can be used to investigate specific features or the distribution of materials. Horizontal probing allows exploration of the subsurface along the length of the alignment of a tunnel or other infrastructure. The use of oriented exploration tools, while common in the energy exploration industry, is less common for civil underground structure development. This may be due to cost, but perhaps also to unfamiliarity with the technique among site investigation professionals. It may be argued that incentives. For efficiency are less evident for engineers than for contractors and owners who realize savings from efficiency.Recent developments in boring technologies include cryogenic drilling capabilities for boring in difficult materials and measurement while drilling (MWD) systems that provide early information on the materials useful for guiding future borings and planning an efficient testing program. The profession has yet to widely adopt these techniques.Noninvasive TechnologiesNoninvasive site characterization tools include remote sensing (e.g., satellite and terrestrial light detection and ranging (LIDAR), digital photogrammetry, radiometric technologies, and interferometry methods) and ground geophysical techniques (e.g., seismic refraction and reflection, spectral analysis of surface waves (SASW), crosshole tomography, geoelectric, electromagnetic, and potential field methods gravity, magnetic) that provide data from which subsurface conditions may be inferred.
Noninvasive techniques are best used in combination with invasive techniques to provide a more complete understanding of underground conditions. Advantages of noninvasive technologies are the speed with which they can be used and that larger volumes of the subsurface can be characterized. Disadvantages are that data generally must be reduced from their raw form—inversion modeling is often required to evaluate ground zonation and materials properties. Such models are non-unique (e.g., a single data set can yield infinite models), and hence special skills and knowledge are required to reduce and interpret data. The cost of some of these methods can also be high, but as the methods become more common and the technologies continue to improve (e.g., laser scanning), the cost of data acquisition and analysis will go down.There is significant opportunity to improve data gathering related to ground properties and the presence and location of existing structures using noninvasive technologies, but there are physical limitations in terms of the scale of objects to be characterized and the material property differences that can be identified relative to the depth of investigation possible. There is, for example, a practical limit for pipe detection using surface-based ground penetrating radar (GPR), reported to be the ratio of approximately 12:1 for the detection depth to the detectable pipe diameter, even under favorable soil conditions (Sterling et al., 2009). This means that a 1-foot diameter pipe can only be detected if within 12 feet of the surface, and a 1-inch pipe can only be detected up to a depth of 1 foot.
Research into the fusion of multi-sensor data that would allow noninvasive technologies to accommodate a wider range of ground conditions and to improve their ability to resolve ground properties and the presence and location of buried objects is under way both in the United States and overseas. Similarly, research by the military into the detection of land mines and deep covert tunnels can have significant benefits in broader civil engineering applications. Characterizing Existing Infrastructure and Legacy Construction MaterialsFailing to locate existing infrastructure before repairing existing or installing new infrastructure is a potential source of accidents.
Legacy construction materials, including unmapped abandoned piles, foundations, or tiebacks that once provided support during previous construction, are regularly encountered during underground construction. Identifying and characterizing these artifacts is a necessary part of site characterization. Historical records found in planning departments can be used to identify locations of some legacy materials, but the records are often incomplete, inaccurate, or missing, thereby necessitating a greater reliance on exploration technologies, especially noninvasive, for characterization.Unmapped or inaccurately mapped underground infrastructure poses potential hazards and risks for underground construction workers, the construction site, other infrastructure, and other people in the vicinity. Encountering unexpected infrastructure may necessitate revised construction planning or repairs. Existing or legacy infrastructure may be avoided, but sometimes must be protected with support designed to avoid displacement or damage to existing or planned infrastructure. The committee notes that every open-cut excavation, bore, or tunnel is an opportunity to assess and document the ground properties and structures encountered for present and future applications.
The hundreds of thousands of open-cut excavations for utility work made every day in the United States, for example, offer repeated opportunities to collect and archive such data. However, the fidelity of nonivinasive techniques to identify subsurface infrastructure needs to be improved. Additionally, investigation technologies need to be integrated with new physical tools and administrative structures to capture this type of information. Mechanisms that allow dynamic archiving (e.g., continuous updating and modification) of these data are critical to the sustainability of urban infrastructure.Interpreting and Integrating Site Characterization DataSite characterization information and data must be processed and evaluated to develop interpretative geologic models and to generate the engineering parameters to be used in underground facility design (see examples in ). Many field techniques used for preliminary property classification have been applied for decades and are subject to gross differences in interpretation. Typically, field classification cannot substitute for laboratory verifications.
Many tools aid interpretation of, for example, rock classification including empirically based procedures such as the Q-system (for rock quality classification) (Barton et al., 1974), the Rock Mass Rating system (Bieniawski, 1976, 1989), and the Geological Strength Index (Hoek, 1994) (see for example classification). Classification schemes for characteristics such as strength and stiffness also are used to establish the input for advanced numerical analysis procedures. BOX 6.1Three-dimensional Geologic ModelingThree-dimensional modeling of ground conditions that incorporate geotechnical and geophysical data is conducted extensively in the United States for development activities including resource exploration and extraction and infrastructure development. Similar modeling techniques can be applied to urban infrastructure planning, risk modeling, and resource management as is done by the British Geological Survey (see ). Such models can provide multiple “views” (e.g., orientations), be “exploded” (e.g., layers can be visually separated to isolate specific features or units), and otherwise manipulated to identify predicted physical properties at depth, the locations of anthropogenic structures, aquifer vulnerabilities, and other qualities. Such comprehensiveFIGURE 1 Example of three-dimensional engineering geological modeling employed by the British Geological Survey for visualizing variability in geologic materials and their physical properties.
SOURCE: Reeves, 2010. Reproduced by permission of the British Geological Survey.
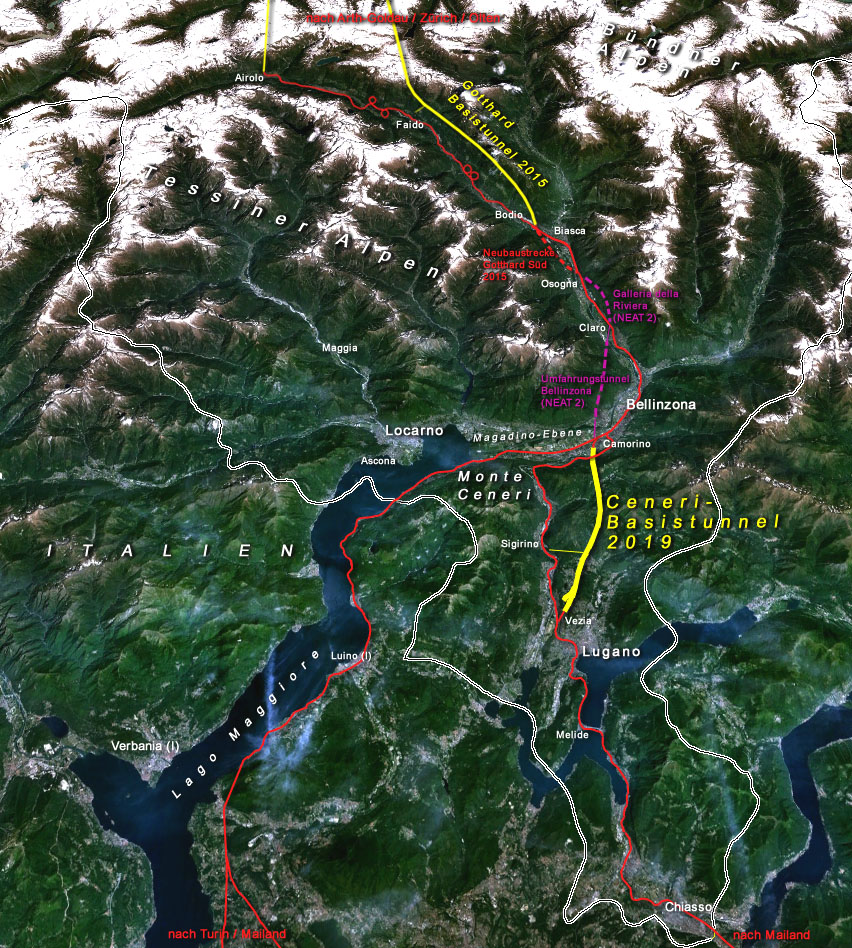
All rights reserved. CP12/073.From a broad perspective, however, insufficient use is made of all the classification and material testing that is carried out on the thousands of individual projects that occur in a medium- to large-sized city every year. Collection and integration of such data remain difficult because, to be useful, the data must be carefully documented and referenced as to location, depth, other properties, and pedigree (e.g., data sources, what tests were run, and was test equipment properly calibrated). Also, and perhaps the most telling, are the significant disincentives for project owners and their consultants to release data because of concerns related to liability and loss of proprietary knowledge. Nevertheless, regulations exist, for example, that require well boring operations to submit their boring logs to state geological surveys. Steps to usefully capture more of the geotechnical.
Views of data can enhance decision making and help to quantify uncertainties that historically are a source of difficulties in contracting and litigation. Confidence maps can be created based on data density and geologic complexity that indicate areas of low or high uncertainty in models (see ).
Given variability of geologic conditions and the spatial limitations of underground characterization tools, information about the underground is often limited and includes significant uncertainties.FIGURE 2 A bedrock confidence map produced for an area in Glasgow, Scotland. The green points represent actual data points; the roughly vertical planes represent faults.
Contours represent levels of uncertainty based on data density and geological complexity of modeled surface (red indicating high uncertainty). Such maps provide valuable insights regarding where more data may be needed. SOURCE: Reeves, 2010. Reproduced by permission of the British Geological Survey. All rights reserved. CP12/073.data generated represent important ways to help enable the sustainability of the urban underground and the regions it serves.To move toward engineering practices that are consistent with such sustainability goals, data related to underground infrastructure development need to be archived in formats and with tools that make them retrievable and accessible for the infrastructure life cycle—and beyond (to account for infrastructure artifacts in place well after closure or decommissioning). These issues will be discussed in a later section related to the critical challenges of archiving infrastructure-related data.

Figure 6.5 Support categories estimated from the tunneling quality index (after Grimstad and Barton, 1993) SOURCE: Palmstrom and Broch, 2006.Sustainability as an integral part of design is a relatively new concept. Sociopolitical and economic issues, discussed in earlier chapters, influence infrastructure design, perhaps at the expense of sustainability.
Design and analysis of underground infrastructure is often heavily focused on the immediate opening and support of underground space; long-term issues related to sustainable maintenance and use are often overlooked, as are lifecycle contributions of the infrastructure to society. Project constraints such as rights of way and access can impact selection of project alignment, and physical constraints such as those associated with water conveyance gravity systems (e.g., slope, number of lift stations, length of drives), maximum grade (for construction and operation), and shaft location also may affect design choices. New technologies or policies that reduce the adverse affects of such physical constraints could reduce the cost of. Developing underground space. As discussed in, codes and standards guide design and operation, especially where public safety is affected. Although building codes can protect the health and safety of those constructing, operating, or using infrastructure, building codes are static in nature and, therefore, can detract from sustainability goals and leave little room for flexibility or evolution of technologies.Constraints created by the limitation of design tools, or by the limitations of those who use the tools, also limit the ability to design underground infrastructure optimally and to reduce lifecycle costs.
Underground infrastructure design can benefit from iterative analyses of designs—especially innovative designs—in a virtual environment. Design performance in difficult ground conditions can be predicted using numerical models, and results of analyses can inform infrastructure design modifications that meet constraints and desired parameters. The process can be repeated as often as necessary until desired model responses and optimal design are achieved. Commercial software packages are available for this sort of analysis, but their use is successful only if their limitations are recognized. Needed in geotechnical design is greater consistency in numerical modeling usage, project design validation, and integration of modeling with other analysis components (scaling) to arrive at more sustainable design. This requires software enhancement, especially with respect to graphical user interface interactions, error checking, and user training. Another challenge is the lack of appropriate ground behavior model input.
Field and laboratory test data are often not appropriate for input in advanced analysis or model verification. Stronger and more direct linkages between field investigation data and model development are needed (e.g., Hashash et al., 2009).The proper combination of technology, training, and skills are vital to good engineering design. The design of sustainable underground facilities is a complex and iterative process that is necessarily a team effort, as described in. Continuous communication between engineers, owners, and interested third parties is necessary to develop optimal designs that meet the owner’s specific requirements and societal sustainability goals.
Input from a broader array of stakeholders helps create infrastructure that is integrated into the system of systems that comprise urban development. Designers need to contend with how the space fits within existing infrastructure, its long-term impacts on the total urban environment, and its resilience over time.The next sections describe typical major inputs to the design of underground projects.An example of this is a Japanese law pertaining to the public right to space of greater than 40 m depth as a means to enhance planning and sustainability of underground resources. The laws allows rational choices for project alignments and preservation of the underground for future uses (Konda, 2003). Integrating Design and AnalysisGood engineering design includes analysis of system performance as part of design development. Many tools are available to design details of underground structures—for example, thickness of supports or appropriate size and location of ventilation shafts. Empirical and analytical design methods, ideas and procedures based on experience, building codes, and analytical and computational software packages all contribute to optimal design of underground structures.
However, their effectiveness directly correlates with the quality of data input and the knowledge base and experience of those applying the tools.ExperienceThe lack of education and training that allows good engineering of underground systems is of growing concern in the United States and will ultimately affect the resilience of underground infrastructure and sustainability of the urban development. Fewer than five U.S. Institutions of higher education provide student opportunities in underground construction, and engineering talent is being imported from overseas. Similarly, it is critical for the underground construction industry to develop mechanisms that allow the relevant transfer of experience from senior personnel to young engineers.Empirical Design ToolsEmpirical design methods rely on quasi-quantitative approaches to material characterization based largely on experience-driven judgments. They are not necessarily based on fundamental mechanisms of ground and structure behavior. For example, soil and rock classification systems are based on the comparison of observed material behaviors from the infrastructure site to similar observations made elsewhere. Behavior is predicted based on those comparisons, but the underlying reasons for the behaviors may not be well understood.
Even so, the soil and rock classifications based on empirical characterization often inform support requirements and design specifications. In some cases, empirical characterization may be based on a large number of prior observations, and resulting conclusions may be robust. In other cases, few data exist to enable comparisons, and empirical characterizations may be more similar to “best guesses.” Empirically based conclusions can be considered first-order estimations, and further observations may be necessary to confirm or refine those estimates. In other words, they can be used to interpolate but not extrapolate. Improper use of empirical tools can result in safety hazards, poor performance, or unnecessary expenses. Educating professionals about the limitations of empirical methods is one way to improve their use. Improving the databases of observations and the methods for their expansion is another.
Analytical and Computational MethodsAnalytical methods—primarily closed-form mathematical solutions that do not require the use of a computer—for calculating stresses, strains, ground movements, groundwater flow, and other properties are critical to improving the understanding of underground structure behavior in concert with the ground environment. They provide data on the responses of underground structures for particular idealized boundary conditions and material behaviors. Analytical methods are key tools for building knowledge and judgment regarding geotechnical behavior, for carrying out preliminary design studies (or full design given suitable configurations), and for comparing complex computational model outputs to gauge reliability. However, they cannot capture the full complexity of most underground structures and geological conditions.Computational methods such as finite element, finite difference, and discrete element methods are used to estimate behaviors of soil, rock, or engineered structures when a sufficient amount of detailed information is available—for example, to refine design based on responses—but can be useful when there is limited prior experience working under specific conditions. Behaviors may be predicted using computer software programs. Computational methods may be used to estimate, for example, structure-ground interactions and deformations, changes in the ground environment (e.g., thermal changes and groundwater flow and contamination), and the propagation of fire and smoke in occupied underground spaces.
BOX 6.2Analytical and Computational Model DevelopmentMore and better data are not enough. Under increasingly difficult ground conditions.
Underground technology development and engineering advances are spurred by underground construction requirements throughout the world (Parriaux et al., 2006), and by the need to remain competitive in an international market for underground construction contracts. The importance of underground infrastructure development that contributes to sustainable development is now being recognized (e.g., ECTP, 2005).Sustainability of the urban environment is partially dependent on minimizing disruptions to economic output of populated areas during construction (and later maintenance) of underground infrastructure.
Underground projects often take years to complete, and during that time equipment is moved to and from an excavation site and huge volumes of excavated soils and rock (muck) must be moved. Streets may be blocked for extended periods, and truck movement on surface streets can be disruptive and polluting. Because each project and environment is unique, no single engineering solution minimizes disruptions, use of nonrenewable resources, and costs for all underground projects. However, technologies are available if there is a willingness to incur additional startup expenses.
FIGURE 6.6 Braced excavation for a cut and cover tunnel section of the Boston Central Artery. Hashash.floors of a building while the basement excavation is continuing. Cut and cover also can be used in submarine tunnels.Major cut-and-cover projects in urban areas provide opportunities to update other underground facilities in their vicinity.
For example, utilidors may be incorporated in transportation projects, and underground pedestrian networks in city center redevelopments (as occurred in both Montreal and Toronto) may be built. The direct and indirect comparative impacts of cut-and-cover construction in the public right-of-way as compared to less intrusive construction approaches such as bored tunnels and trenchless technologies are not always well enough understood to inform decision making.Tunneling and Ground Support Technologies in RockRock excavation is required for all types of underground uses including mountain tunnels, mining operations, and large underground caverns where rock is at or near the surface. In historic times, rock excavation was painfully slow, relying on hand tools or even thermal shock to disintegrate the rock. The introduction of blasting technologies in the sixteenth and seventeenth centuries revolutionized the speed of rock excavation and increased the range of projects that could be undertaken cost-effectively. In the past half-century, hard-rock TBMs have found widespread application creating medium length to long tunnels. RockFires were used to heat rocks, which were then quickly cooled with water. The rapid change in temperature caused rocks to crack and break.
Broken rock could then be excavated. Excavation also benefited from the emergence of rock mechanics as a distinct branch of geotechnical engineering in the 1950s. Study of rock fragmentation, understanding of the post-peak strength behavior of rocks, and characterization of the influence of rock discontinuities on rock mass behavior are among the important outcomes from research in rock mechanics.Drill and Blast TechnologiesIn drill and blast excavation, explosives and timed detonators are placed into drilled holes, the blast is carried out, the opening is ventilated, waste spoil is transported away from the excavation face, and the process starts again until the desired opening is obtained (e.g., Sellers et al., 2010). Blasting is typically the preferred solution from a cost perspective for short rock tunnels and for the excavation of foundations and rock caverns. Blasting, however, can be especially challenging in urban environments given higher amplitude vibrations (and therefore, ground shaking), larger sized spoil material, and a greater need for primary tunnel support than, for example, that result from TBM use.
Excavation speeds are relatively slow, the process is noisy, and overbreak (excavation of too much material) is possible.Alternatives to conventional drill and blast technologies are possible. For example, propagating fractures from boreholes in rock using controlled gas expansion could allow continuous excavation without releasing the excess energy that causes vibration and flying rock. The commercial application of such technologies so far has been restricted to specialized applications where conventional blasting is not feasible.Blast design (e.g., blast patterns and sequences) could be more effective with improved computational models of drilling, blasting, vibrations, and displacements. Designs that minimize noise and vibration, for example, may allow blasting at any time of day, increasing productivity, minimizing disruptions to local neighborhoods, and minimizing damage to surrounding infrastructure. As cities become more densely developed and use more underground space, blasting approaches that are proven to minimize damage and nuisance will be desired.Tunnel Boring Machine Technologies for Rock ExcavationEarly variations of TBMs for soft rock were developed in the late 1800s (e.g., the Beaumont English boring machine was used on one of the early attempts to create the Channel Tunnel between England and France; Maidl et al., 2008). Early advances in TBM design were made by the Robbins Company of Seattle, Washington, which remained the world-wide leader in TBM design and manufacture for many decades. During the past decade, however, TBM manufacture has become increasingly dominated by non-U.S.
Companies.Machine tunneling in harder rock remained a challenge because of inefficiencies and rapid wear of the pick-type rock cutters in use at the time. With the advent of full-face machine design and disc cutters for rock and proof of the. Value of the TBM approach (e.g., the Chicago Tunnel and Reservoir Plan), the hard-rock TBM using disk cutters gradually supplanted drill and blast technologies for longer tunnels.Advance rates using TBMs in soft rock can exceed 100 ft per day. However, tunnel excavation is a systematic and industrial production process that can only proceed at the speed of the slowest element in the system. Thus, technological advances that increased the speed of rock fragmentation could not be fully applied until the muck conveying systems that remove excavated materials could be designed to keep pace. Advance rates for rock excavation also could be improved with more efficient methods of installing supports and reinforcements. Ground control measures to prevent loss of confinement and therefore face instability, chimneys, cutterhead blockage, and tunnel collapse in blocky and highly fractured ground could be improved.
Improved methods for predicting and mitigating spontaneous, energetic, and sometimes hazardous rock fracture (rock-bursts) in highly stressed competent rock could minimize risk and result in more repeatable construction practices, more consistent work products, and better control of construction times and costs. This parallels a need for fundamental advancements in our understanding of rock mechanics and rock behavior, including three-dimensional rock fracture mechanics.With better quantification of rock and excavation tool interactions, there can be increased use of systems that sense their own progress and functioning (smart systems), more automation in underground construction (e.g., robotics) to reduce risk to workers, and enhanced ability to probe ahead of the excavated face to detect changes in material properties.
Modern TBMs are computer-controlled, high-tech machines that use laser guidance systems and sensors to obtain realtime information on system performance. Data obtained during excavation can be used in feedback loops to optimally adjust, for example, thrust and rotation speeds.Tunneling and Ground Support Technologies in SoilIndurated rock may be more difficult to fragment while tunneling, but tunneling in soils (unconsolidated materials) presents challenges related to ground support, muck removal, and excavation-induced effects on the surrounding ground (e.g., ground settlement, lowering of the groundwater table). Improving control of deformation due to tunneling in soil is a key to limiting damage to existing infrastructure and improving capacity to sustainably develop underground space in most urban areas.Tunnel lining is also an area where efficiencies can promote sustainability.
Costs, for example, may be reduced when initial and permanent support (e.g.,Vertical openings from the tunnel to the surface created as a result of displacement of material into the tunnel. Construction support and final lining) are integrated, or when thicknesses of linings are reduced through use of new higher-strength materials. Other beneficial advances could include enhancing techniques to decrease overcut during mining, improving directional control during mining to decrease the allowances for alignment errors, and developing more efficient and rapidly deployed formwork for cast-in-place linings.Tunnel Boring Machines in SoilRecent technological developments for tunneling in soil include the earth pressure balanced TBM (see ) and the slurry shield TBM (Figure 6.3).
These simultaneously excavate and support a tunnel face by creating a separate chamber (bulkhead) that closes off the face from the rest of the tunnel. These advanced technologies greatly mitigate risks of tunneling in saturated soils including those associated with running soils at the face, significant settlement in poor ground, and excessive water pressure. In the past, machine tunneling was not possible for deep tunnels under high water pressures without the use of compressed air in the tunnel—an expensive process with significant safety and risk issues. Comparatively, the earth pressure and slurry technologies greatly minimize settlement and associated damage, increase excavation speeds, and reduce costs. As a result, increasingly larger tunnels have become safe and economical to construct in poor soil conditions and allow such tunneling to contribute to more sustainable solutions for transportation and other infrastructure needs.Sequential Excavation MethodsSequential excavation methods (SEM) include multiple applications such as the New Austrian Tunneling Method and the Sprayed Concrete Lining Method that rely on integrating the excavated rock or soil into the tunnels’ supporting structure (FHWA, 2009).
SEM design includes deliberately relieving stress by mobilizing the ground around a tunnel to the maximum extent possible through controlled deformation. The methods involve commitment to both a design philosophy and a construction method largely dependent on the observational method. Initial primary support of the tunnel is designed with consideration for load-deformation characteristics appropriate to existing ground conditions; the required installation speed is based on the magnitude and speed at which ground deformations develop. The primary lining provides initial support during construction, preventing roof collapse. Instrumentation is installed to monitor deformations in the initial support system and to inform of changes needed in the support design and excavation sequence. The tunnel is sequentially excavated and supported, and the excavation sequences can be varied.
The permanent support is usually (but not always) a cast-in-place concrete lining. SEM can be applied in both shallow and deep excavations, but in either case the effects of deformation on surrounding structures and the surface need to be considered.Ground support in SEM can be selected or optimized in real time to match. The ground conditions uncovered during excavation. This feature is intended to support the least favorable conditions encountered in the alignment and is in contrast to a pre-selected support system that does not accommodate variation in conditions along an alignment. The amount and the cost of materials needed for ground support can thus be reduced. However, the approach has its limitations: continuously changing the support scheme may reduce construction crew productivity and delay project construction.
Further, the decision processes used in SEM are dependent on information that is not readily available (e.g., as related to ground condition, ground response, and movement). Research is needed in these areas to improve SEM application and contribution to more sustainable underground space development.Trenchless TechnologiesMany new underground utility construction and repair technologies have emerged in the past 40 years that allow installation, replacement, or repair of underground utilities or conduits without excavating a continuous trench from the surface.
Although “trenchless” also applies to larger bored tunnels, the term typically refers to urban-utility-scale technologies rather than to rail, metro, or road tunnel installations. Trenchless technologies introduce new solutions for minimizing surface disruptions into short and long-term planning, design, and operation of underground systems. This will be especially true as the techniques become more commonly used.Descriptions of trenchless technologies can be found in books and reports (e.g., Kramer et al., 1992; Thompson, 1993; Stein, 2002, 2005; Najafi and Gokhale, 2005), multiple conference proceedings (e.g., ISTT, 2010), journals (e.g., Broch et al., 1986), and magazines., Figure 6.7 illustrates major trenchless technologies and applications. Because many technological advancements have centered on better equipment or new processes, complete understanding of the processes from a design and application perspective has lagged behind adoption in the field. Much information about the technologies is obtained directly from installation contractors, manufacturers, suppliers, or through trade associations. This potential lack of communication between engineering designers and construction contractors is problematic.
In addition, the relatively new ability to install utilities at low cost beneath existing shallow utilities without major disruption to the utilities or traffic provides the ability—and even incentive—to deepen the existing spaghetti layout of utilities as pictured in Figure 1.6. However, this could interfere with future placement of major transportation or water and sewer infrastructure. In other words, such construction technologies heighten the needTrenchless Technology, magazine published by Benjamin Media, Peninsula, Ohio.Underground Construction, magazine published by Oildom Publishing, Houston, Texas. FIGURE 6.7 Slurry shield machine for the East Side Access Project, Queens, New York. (left) Assembly of two tunneling boring machines (TBM) and their trailing gear in the launch pit. (right) Closeup of the cutter head of the TBM.
Hashash.to address long-term planning and the ability to choose the most sustainable and best uses of urban underground space.Ground Improvement TechnologiesIt is often necessary or productive to temporarily or permanently change soil or groundwater properties during underground construction to ease facility design, construction, or operation. For example, ground freezing can temporarily turn a weak, saturated soil into a solid and nearly impermeable material; dewatering can ease construction problems both in terms of water flow and soil stability; and grouting can be used to stiffen or change the permeability of soils. Ground modification techniques and the development of new materials used for geoenvironmental and geomechanical applications (e.g., bio- and nanotechnologies; NRC, 2006) are active areas of research and field application. Furthering application of established methods (e.g., jet grouting and compensation grouting), as well as developing new approaches and materials, offer the potential for cost reductions and performance improvement. However, as for trenchless technologies, application of new ground improvement technologies may outstrip theoretical understanding of the methods, meaning that application of these techniques in design may not be optimized.
Further research and development is needed to improve existing methods, enhance understanding of their application, and allow better engineering with, for example, in situ biotechnologies, nanotechnologies, and other ground improvement and remediation techniques that can reduce the.
Tunnels - Brian Williams SummaryFourteen-year-old Will doesn't think he has much in common with his family. Nothing, that is, except a strange passion for digging which he shares with his father.
Underground Bases And Tunnels What Is The Government Trying To Hide Pdf
But one day, Will's dad mysteriously vanishes down a tunnel - part of London's vast, labyrinthine underground system. With his friend Chester, Will decides to investigate. But soon the boys find themselves deep in darkness, unearthing a terrifying secret which may cost them their lives. This riveting bestseller oozes the mysterious fascination of the underground and all its hideous possibilities. Creepy, tantalising and original, it's full of thrills to keep you burrowing in! Deeper - Brian Williams SummaryThe gripping sequel to Tunnels. Will is going deeper under the earth.
Deeper into horror, heat and darkness. Every step takes him deeper into mystery. Deeper into terrible danger. As Will, Cal and Chester venture ever further into the Deeps, they enter a place where those banished from the Colony stand almost no chance of survival. Battling heat and deadly prehistoric creatures, they tunnel through caves and deserts - but are they any closer to finding Will's father? And now that the Styx are on their tail, what chance do they have of completing their foolhardy mission? Design and Construction of Tunnels - Pietro Lunardi SummaryThis work illustrates how the Analysis of Controlled Deformation in Rocks and Soils (ADECO-RS) is used in the design and the construction of tunnels.
This is a very new and effective way of tunnel construction. The ADECO-RS approach makes a clear distinction between the design and the construction stages and allows reliable forecasts of construction times and costs to be made. It uses the advance core (the core of ground ahead of the face) as a structural tool for the long and short term stabilisation of tunnels, after its rigidity has first been regulated using conservation techniques. AUA Guidelines for Backfilling and Contact Grouting of Tunnels and Shafts - Raymond W.
Henn,American Underground Construction Association. Technical Committee on Backfilling and Contact Grouting of Tunnels and Shafts Summary- Introduction - Affects of geological conditions of grouting - Structural and operations requirements of the completed facility - Grouting of various lining types - Grout materials - Grout properties - Backfill grouting - Contact grouting - Grouting equipment - Record keeping - Quality control - Contract documents. Sprayed Concrete Lined Tunnels - Alun Thomas SummaryPractising engineers on site, in the design office or in client organizations will find this book an excellent introduction to the design and construction of sprayed concrete lined (SCL) tunnels. The complex behaviour of the early age behaviour of the sprayed concrete requires careful management. This book covers all aspects of SCL tunnelling – from the constituents of sprayed concrete to detailed design and management during construction. Although there is a close interdependence between all the facets of sprayed concrete, few engineers have the right breadth of experience and expertise, and this urgently needs to be transferred to the wider engineering community.
Disseminating essential information for tunnelling engineers, Sprayed Concrete Lined Tunnels is key reading for all involved in or studying the process. Risk Assessment and Security for Pipelines, Tunnels, and Underground Rail and Transit Operations - Anna M. Doro-on SummaryRisk Assessment and Security for Pipelines, Tunnels, and Underground Rail and Transit Operations details a quantitative risk assessment methodology for systematically analyzing various alternatives for protecting underground rail, oil and gas pipelines, pipeline freight transportation, and other tunnel systems from terrorism threats and other disasters.
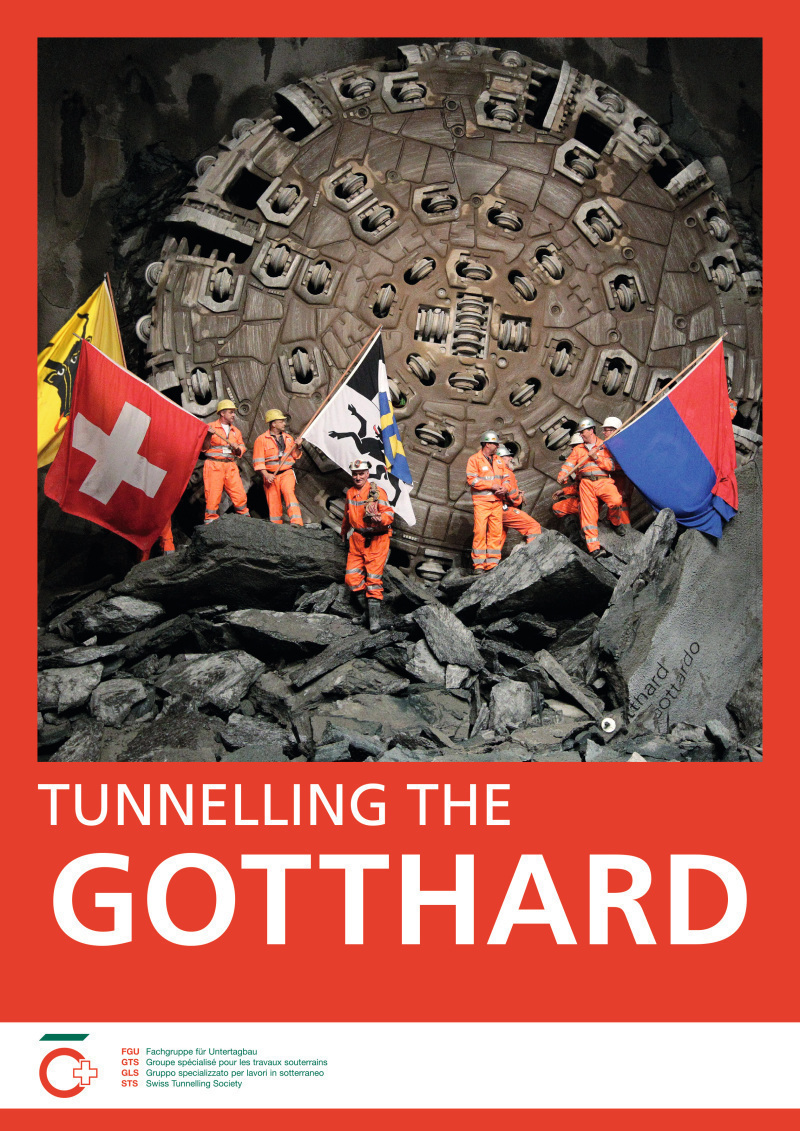
It examines the engineering, environmental, and economic impacts and addresses both direct and collateral damage. The book describes how to employ the methodology of quantitative psychology for effectively assessing risk in homeland security, defense actions, and critical infrastructure protection. Using pipelines, tunnels, underground rapid rail, and transit systems as examples, it maintains an emphasis on applying quantitative psychology to risk management in the areas of homeland security and defense. Outlines the background and system operations of pipelines, tunnels, underground rail, and transit systems as well as other super-speed futuristic trains Covers materials used for fabricating weapons of mass destruction and operations for terrorism Deals with the probabilistic risk estimation process, event tree analysis, and fault tree analysis Discusses the risk and vulnerability assessment tools and methodologies used by experts and governmental agencies Approved for public release by the U.S. Federal Government, this book presents regulations, standard processes, and risk assessment models recommended by the U.S.
Department of Homeland Security and other federal and state agencies. Describing how to evaluate terrorism threats and warnings, it details protocols for preventive measures and emergency preparedness plans that are based on economic analysis. With comprehensive coverage that includes risk estimation and risk acceptability analysis, the book provides a foundational understanding of risk and the various defensive systems that can improve safety and security as well as thwart terrorists’ efforts to sabotage critical infrastructure. Immersed Tunnels - Richard Lunniss,Jonathan Baber SummaryImmersed tunnels have been around for more than a century but remain a relatively unknown form of tunnel construction.
For waterway crossings they are an effective alternative to bored tunnels and bridges, particularly in shallower waters, soft alluvial soils, and earthquake-prone areas. Successful implementation requires a thorough understanding of a wide variety of civil engineering disciplines and construction techniques. Immersed Tunnels brings together in one volume all aspects of immersed tunnels from initial feasibility and planning, through design and construction, to operation and maintenance. Get Valuable Insights into Immersed Tunnel Engineering from Expert Practitioners The book presents design and construction principles to give a full appreciation not only of what is involved in an immersed tunnel scheme but also how potential problems are dealt with and overcome. It examines important factors that have to be considered, particularly environmental implications and mechanical and electrical systems. It also gives practical examples of how specific techniques have been used in various projects and highlights issues that designers and constructors should be aware of. In addition, the book discusses operation and maintenance and reviews contractual matters.
These aspects are described from the viewpoint of two experienced practitioners in the field who have a wealth of experience on immersed tunnel projects worldwide. As tunnels are increasingly being adopted as engineering solutions around the world, this unique and extensively illustrated reference explores the wide variety of immersed tunnel techniques available to designers and constructors. It provides essential insight for anyone involved, or seeking to be involved, with immersed tunnel projects. Technical Manual for Design and Construction of Road Tunnels-civil Elements - N.A Summary'The increased use of underground space for transportation systems and the increasing complexity and constraints of constructing and maintaining above ground transportation infrastructure have prompted the need to develop this technical manual. This FHWA manual is intended to be a single-source technical manual providing guidelines for planning, design, construction and rehabilitation of road tunnels, and encompasses various types of road tunnels'-P.
Fire and Rescue Service operational guidance - incidents in tunnels and underground structures - Great Britain: Department for Communities and Local Government,Chief Fire & Rescue Adviser SummaryThis publication presents a framework for a safe system of work for operations at incidents involving tunnels and underground structures. It provides a robust yet flexible insight that can be adapted to the nature, scale and requirements of the incident. Incidents involving tunnels and underground structures may place significant demands on local fire and rescue services and have often required a national co-ordinated response from across the country. This type of incident may generate intense media attention where the operations of the emergency services are rigorously scrutinised. Whilst much of this attention is approving it will invariably focus on the preparedness of the emergency services and their operational effectiveness.